Basic sciences in reconstruction of the anterior cruciate ligament with the ischiotibial tendons (a narrative review)
Ciencias básicas en la reconstrucción del ligamento cruzado anterior mediante los tendones isquiotibiales (revisión narrativa)
Resumen:
El resultado final de la cirugía reconstructiva del ligamento cruzado anterior (LCA) depende en parte del sumatorio de pequeñas decisiones durante la preparación del injerto tendinoso que sustituirá al LCA. Los tendones isquiotibiales autólogos son frecuentemente utilizados como injerto para la sustitución del LCA.
Atendiendo a sus propiedades reológicas, el injerto de LCA durante su manipulación (previamente o tras su colocación) debe estirarse dentro de su comportamiento elástico para mejorar las condiciones de tensión que se esperan de él tras la cirugía. Bajo este punto de vista, las referencias isométricas pueden resultar más seguras para evitar el estiramiento excesivo de la plastia.
Una vez extraídos los tendones, no es factible construir un injerto largo y grueso al mismo tiempo. Además, hay una imprevisible variabilidad anatómica tanto en las longitudes como en los diámetros de los tendones extraídos. Por esa razón, los tendones se manipulan mediante plegados y suturas hasta obtener la configuración de injerto adecuado. Plegar en 4 el semitendinoso (4ST), doblarlo en 2 y asociar el mismo gesto con el gracilis (2ST-2G), triplicar alguno de ellos o separarlos en 2 fascículos exigen diferentes manipulaciones. No está avalada la presencia de los tendones suficientes para cualquier talla o condición de riesgo de los/as pacientes.
El injerto, finalmente, deber ser fijado biológicamente en el hueso receptor. El hábito quirúrgico de considerar suficiente un túnel de 15 mm ha sido inferido desde estudios biomecánicos experimentales con animales. No ha sido demostrado en humanos y debe interpretarse con cautela.
Abstract:
The final outcome of surgical reconstruction of the anterior cruciate ligament (ACL) is partially dependent upon the sum of a number of small decisions during preparation of the tendon graft that will replace the ACL. Autologous ischiotibial tendons are often used as grafts for ACL substitution.
Based on its rheological properties, the ACL graft must be stretched within its elastic behavior range during manipulation (before or after placement) in order to improve the tension conditions expected of it after surgery. From this perspective, isometric references may be safer for avoiding excessive stretching of the plasty.
Once the tendons have been harvested, it is not feasible to construct a graft that is long and thick at the same time. Furthermore, there is unpredictable anatomical variability in both the length and the diameter of the harvested tendons. For this reason the tendons are manipulated through folding and sutures until an adequate graft configuration has been obtained. Folding the semitendinosus in four (4ST), doubling it in two and combining the same maneuver with the gracilis (2ST-2G), tripling some of them or separating them into two fascicles, require different manipulations. The availability of sufficient tendons for any size or risk condition of the patients is not guaranteed.
Lastly, the graft must be biologically fixed in the receptor bone. The surgical habit of considering a 15 mm tunnel to be enough has been established from experimental biomechanical studies in animals. It has not been demonstrated in humans and must be interpreted with caution.
Introduction
Autologous biological substitutes are used for reconstruction of the anterior cruciate ligament (ACL). Grafts involving the tendons of the medial ischiotibial muscles are often used due to the low morbidity of the harvesting site compared with the patellar tendon(1).
Most published studies focus on technical aspects (surgical reconstruction models, fixation devices, etc.) or on the specific clinical outcomes obtained. Studies on the biomechanical characteristics of ischiotibial tendon grafts are less numerous and lack a transverse perspective.
The length or diameter of the tendons, their different forms of folding or cohesion with sutures for configuration of the plasty, their possible prior traction, etc., are different yet related variables.
The surgeon must have a transverse view in order to handle the sum of many small decisions during surgery that affect the final outcome. The present review analyzes the physical and biological characteristics of the ischiotibial tendons and their implication in reconstruction of the ACL, from a basic science perspective.
Rheological properties of the tendons
Static analysis
Tendons are biological materials with anisotropic physical properties, i.e., their properties are dependent upon the direction in which they are examined (resistance up to 1000 times greater in traction testing along the long axis versus the transverse axis)(2).
The "stress-deformity" plots of collagen-rich tissues can be divided into three regions: 1) the initial region (toe region); 2) the linear region (elastic behavior); and 3) the yielding and failure region (plastic behavior) (Figure 1).
reacae.29276.fs2207013-figure1.png
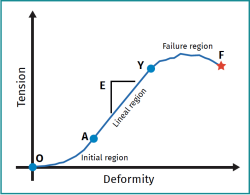
Figure 1. Stress-deformation curve. 1) Initial region (toe region): the curve describes the behavior of the tendons under low deformation (up to 3%), with interweaving of the collagen fibers. 2) Linear region (elastic behavior): the tendon deforms in a linear manner due to intermolecular sliding of the triple helixes of the collagen. If deformation is less than 5%, it proves reversible. 3) Yielding and failure region (plastic behavior).
In the first region, the curve describes the behavior of the tendons under low deformation (up to 3%). It has been seen that the collagen fibrils interweave when the tendons are under resting conditions. As deformation increases towards the linear region, this interweaving disappears and the collagen bundles are stretched. The lack of waviness of the fibrils is considered to be the main cause of the nonlinear relationship between stress and deformation in the initial region.
In the second region, the tendon deforms in a linear manner due to intermolecular sliding of the triple helixes of the collagen. It affords between 3-5% of its elongation, and the deformation is reversible. If the deformation is less than 5%, the tendon will return to its original length once loading ceases. The plot is linear, and the elasticity modulus (slope) is constant (Young's modulus).
Aging has a significant impact upon the mechanical properties of the tendons. Young's modulus of the human patellar tendon at the age of 29-50 years is about 660 ± 266 MPa, but decreases to about 504 ± 222 MPa in the 64-93 years age range(3).
In the third region, the tendon is stretched beyond its physiological limit, and some fibrils begin to fail. The microfailures accumulate, rigidity decreases, and the tendon begins to fail. This happens when the intramolecular cross-bonds between the collagen fibers fail. The tendon thus suffers irreversible plastic deformation. Beyond a lengthening of 5%, it exhibits an irreversible plastic behavior(4). If the tendon is stretched by more than 8-10% of its original length, macroscopic (gross) failure results.
It also should be noted that collagen-rich tissues such as the tendons are viscoelastic materials (i.e., deformation is influenced both by the velocity with which the deforming stress acts and by the duration of such stress). Viscoelastic materials have three main characteristics: flow, stress relaxation and hysteresis. Viscoelastic flow is the permanent deformation that increases with the duration of exposure to loading (Figure 2). This contrasts with the usual elastic material, which does not elongate, independently of the duration of loading. Stress relaxation (Figure 3) in turn is a characteristic of a ligament or tendon, whereby the stress acting upon it ends up decreasing under constant deformation. Lastly, hysteresis has no repercussion in the context of the grafts used for reconstruction of the ACL.
Dynamic analysis
Material fatigue is a phenomenon whereby rupture of the material under cyclic dynamic loading occurs more easily than with static loading. Experimentally, the material is subjected to cyclic loading to a certain stress level (below the static resistance of the material), and the number of cycles to the point of failure is recorded. The behavior of the material is reflected by an S-N plot, where S refers to the applied stress and N is the number of cycles at each particular stress level (Figure 4).
Isolated tendons subjected to cyclic traction loads experience a gradual increase in residual lengthening after the first loading and relaxation cycles. This time-dependent phenomenon is referred to as conditioning, and results from the aforementioned tendon flow properties(5,6). For a given stress level, most tendons reach a stable lengthening state after 10-20 loading cycles. However, if the maximum load is increased in a posterior cycle, the stationary state becomes disrupted and additional loading cycles will be required to establish a new stability state.
Isometry and biomechanical properties of plasties
Strictly speaking, there is no such thing as an isometric graft, defined as that in which the distance between the femoral and tibial fixations remains constant during the range of motion (ROM) of the knee. At most, the concept could apply to a single central fiber within a cylindrical graft. In fact, most fibers of the ACL join to the bone at a certain distance from the axis of rotation, and change in length by various millimeters during the ROM(7).
The concept of isometric behavior of the plasty has been accepted, assuming changes in plasty length of less than 2-3 mm over the ROM(8,9,10,11). It has been seen that the most isometric point of the femur (with less than 1 mm of change in length) is located 3 mm distal to the posterior margin of the Blumensaat (intercondylar) line. It is found in the 10:30-11:00 o'clock position of right-side knees, at the anteroproximal margin of the anatomical fixation of the ACL(12,13) (Figure 5). It approximately corresponds to part of the anteromedial (AM) bundle of the ACL(14). Not only the mentioned classical biomechanical studies of the 20th century but also more recently published studies suggest positioning the graft in the proximal zone of the fingerprint(15,16,17).
On the other hand, there is no fixed axis of motion in the sagittal plane in the human knee. Actually, the rotation axis moves following a descendent trajectory from the position of extension of the flexed knee(18), and this factor may increase lengthening of the graft over the elastic properties.
The single-bundle transtibial technique with isometric references was proposed in the 1990s as a rapid and reproducible procedure. However, residual instabilities were commonly observed in a proportion of these reconstructions of the ACL (of rotational predominance). In order to avoid residual instabilities, the concept of "anatomical" single-bundle reconstruction of the ACL was proposed(19). These "anatomical" single-bundle reconstruction procedures (in a more distal position) have moved away from the isometric reference, however(20). Conceptually, the further away from the isometric zone, the greater stretching will be. Different combinations between the fixations of the tibia and the femur (including both located within the fingerprint) can lead to stretching of up to 10 mm(16,17).
After choosing the location of the tunnel, the surgeon determines the length of the plasty selecting the flexion moment for fixation, thus establishing the graft stress pattern. The mean variation in graft stress during flexion of the knees is shown in Figure 6. In general, all reconstructions show an increase in graft stress as the knee moves toward full extension. The stress in isometric grafts, located posterior and proximal, remains low from 20º to 130º of knee flexion(13). In contrast, the stress levels in grafts placed anteriorly reached significantly higher values than in the more isometric grafts (posterior and proximal) when the knee was flexed 80-120°(13).
reacae.29276.fs2207013-figure6.png
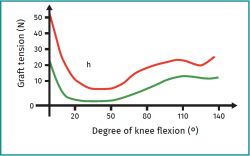
Figure 6. Mean graft stress variations. The green line represents the stress variations suffered by the graft during the range of motion of the knee under "isometric behavior" conditions. The red line represents the stress pattern experienced by the graft with anterior location of the femoral tunnel, presenting significantly increased values in the range of motion of 80-120º.
It can be deduced that if for example a graft is fixed when presenting its shortest distance in extension, it will be exposed to excessive stress during flexion. As materials, tendon grafts stretch irreversibly if their stress cycles exceed 4-5%(3,21). Theoretically, a graft measuring 30 mm in length can only stretch 1.2 mm before stretch becomes permanent. Consequently, moving away from the isometric references during reconstruction could cause the dynamic conditions to lengthen the graft permanently.
Isometric analysis may question the so-called "anatomical" single-bundle reconstruction model(17). A priori, it is not feasible for the graft fibers to exhibit AM and posterolateral behavior at the same time (Figure 7). The more distal the femoral insertion, the fewer fibers with AM behavior will be found in the plasty. Although initial stabilization of the knee is clear, such moving away from the isometric references could be the reason behind the increased number of revision surgeries seen in these reconstructions in comparison with double-bundle reconstructions(22) and even single-bundle transtibial reconstructions(23).
reacae.29276.fs2207013-figure7.png
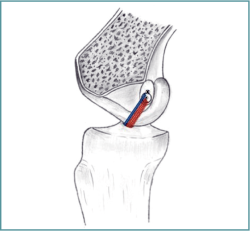
Figure 7. The graft fibers in a right knee cannot simultaneously exhibit anteromedial (blue fibers) and posterolateral behavior (red fibers). Moving the center of the tunnel distal recruits more stressed fibers with posterolateral behavior (stress in extension), though at the expense of reducing the number of fibers available for anteromedial behavior (stress in flexion).
It must be made clear that reaching the point of the femur selected for the femoral tunnel (transtibial or through the accessory portal) and the biomechanical properties of the reconstructions are separate issues.
Diameter and length of the plasty
Ischiotibial autografts have become increasingly popular for reconstructions of the ligaments of the knee. Once the tendons have been harvested, it is not feasible to construct a graft that is long and thick at the same time. According to the reviewed literature, no studies have determined whether it is best to prioritize graft diameter over length. Likewise, it has not been established whether it is better in terms of efficacy to concentrate the available graft tissue into a single bundle or in two bundles. Nevertheless, lower revision surgery rates have been reported in double-bundle ACL reconstructions(22). Preoperative knowledge of the length and diameter of the ischiotibial graft could help the surgeon in deciding how to prepare the graft or choose the model of reconstruction(24). It may be added that the rheological properties of the tendons are not affected by the use of vancomycin during their handling(25).
Traditionally, it has been widely accepted to use quadruple ischiotibial autografts - folding in two both the semitendinosus (ST) and the gracilis (G) in 2ST-2G format - as a successful option for reconstruction of the ACL(26). In order to reduce morbidity in the donor zone and increase the diameter of the graft, use of only the ST tendon in a four-bundle graft (4ST)(as quadruple graft) has been proposed(27,28).
At present, priority has focused on diameter, because many authors have recommended a diameter of over 8 mm in order to reduce the risk of graft failure in single-bundle reconstructions(29,30). In some patients, the aim of securing a graft diameter of 8 mm in ischiotibial tendons can be achieved using a 2ST-2G graft. However, in other patients it may be necessary to add additional ischiotibial tendon graft bundles in order to increase the diameter of the plasty.
Consensus has not been reached in the literature regarding the prediction of graft size. It has been reported that the preoperative anthropometric parameters, particularly patient thigh length and height, are correlated to the length of the ischiotibial graft(31,32,33). Height and weight are considered to be moderate predictors (more reliable in male patients) of the suitability of ST tendon length (in both 2ST-2G format and 4ST format)(34). However, no statistical association has been established between height and the final diameter of the graft(32,34). In no way should the anthropometric parameters be regarded as mathematical equations of predictive value(33).
From the technical point of view, various current surgical options are based on the 4ST format(35). In order to secure optimum construction of the 7 cm quadrupled graft (2 cm in the femoral tunnel, 3 cm intraarticular and 2 cm in the tibial tunnel), we need a minimum tendon length of 28 cm. According to different studies, in at least one of every 5 patients, the length of the ST tendon harvested with the usual procedure tends to be inadequate for use with the 4ST technique (especially in females)(34,36). Considering the above, some ACL reconstruction techniques cannot be applied to all patients due to inadequate tendon length, and would require the surgeon to have some other kind of graft.
With regard to graft diameter, Ramkumar et al. showed that over half of the ischiotibial autografts (in 1681 patients) did not reach the ideal size for reconstruction of the ACL(37). The literature has reported a lesser ischiotibial tendon diameter in female patients(38). The surgeon is unable to guarantee a theoretically ideal reconstruction from either the perspective of graft diameter or in terms of the length of the graft(33). The graft tissue would be insufficient for adequate reconstruction especially in women and in patients of low height.
The literature has also reported that female athletes, young patients of low height, or both conditions simultaneously, are predictors of failure of anatomical single-bundle reconstructions of the ACL(39,40). In this context, the surgeon must be prepared for decision making during surgery. The addition of further bundles must be considered, as well as an increase in diameter using allograft, or the choice of an alternative autograft. From a different perspective, another option would be to choose a model of reconstruction complementing the central stabilizing element with another specific element against rotational instability(41,42).
Configuration of the plasty
The development of both adjustable cortical-support suspensory devices (in femur as well as tibia)(43) and of models of reconstruction (all-inside) that condition plasty preparation have resulted in new models of plasty configuration. The ACL graft comprises a closed tendon loop (often in 4ST format) that must be fixed with sutures(44,45).
In some cases, due to technical defects or because of natural anatomical factors, the harvested tendon might not be long enough to create a 4ST graft of adequate length and diameter. The surgeon then must use alternative resources to gain length. A common practice in this regard is to perform a plasty in 3ST format (gaining length at the expense of a lesser diameter). To gain diameter, the option is to increase the number of plasty fibers with a 3ST + 2G format or even 3ST + 3G format(46). In any case, the surgeon (consuming surgery time) must prepare the plasty, making use of different folding and plasty fiber linking techniques with suture filaments. The aim is to obtain a tubularized and compact plasty that is safe for exposure to the mechanical demands of the knee.
Normally, the Krackow suture is the reference point for traction upon the extremities of the tendons (Figure 8A)(47) during preparation of the graft. It presents differences in mechanical behavior and safety according to the size of the suture, the number of closing loops, and the number of fibers. The sutures are more resistant than the initial mechanical demands of a plasty during its preparation and placement (normally between 60-100 N).
In general, and although any configuration of the suture at the extremity of the tendon may be valid(48), repeated passing of the needle through the extremity (with the laceration this implies) makes this end of the sutured plasty a weak point in the reconstruction(49). This circumstance has led to the development of systems for manipulating the extremities of the plasty that produce fewer perforations in the tendon (potential graft failure site) (Figure 8B) and may even avoid such situations, in addition to allowing for faster preparation times(48).
The 4ST configuration of the plasty (if sufficient length is available) can be used in both the conventional outside-in technique and in the all-inside approach. From the biomechanical perspective, this plasty model affords greater resistance before rupture than the 2ST + 2G plasty(50). The two extremities of the tendon can be manipulated together or separately during 4ST preparation, since both modalities have resistances that exceed the mechanical demands of the plasty during its preparation and placement(51). During preparation of the graft, anchoring the threads of the free extremities of the tendons around the loop of the adjustable devices is biomechanically superior to only anchoring the fibers with transverse stitches (Figure 9)(52). The length of the tendon is a limiting factor for choosing the 4ST configuration. There is abundant scientific evidence that the available length, even in the event of technically optimum harvesting, is not enough to achieve an adequate diameter or optimum length of the plasty within the bone tunnels. Furthermore, there is concern that excess suture envelopment may reduce tendon-to-bone healing in the bone tunnel, thereby adversely affecting biological integration of the graft(53).
The 2ST + 2G configuration (ST and G folded in half and with the extremities free) has coexisted with all the fixation modalities. This includes from interferential screws to current devices adjustable to the length of the tunnel, and also intra-tunnel transfixing fixations. The outcomes remain validated to date(54). This plasty configuration is the option that best guarantees the necessary length in most cases, but not the diameter - as has been mentioned above. From the resistance perspective, its resistance to rupture is even greater than that of the 4ST format. However, permanent elongation in response to cyclic loads is far greater than with 4ST under laboratory conditions(50). The surgeons that choose 2ST + 2G and interferential screws as fixation should be aware that continuous suture of the plasty, under laboratory conditions, affords greater resistance to rupture, though with a decrease in biological fixation surface. Furthermore, when the two tendons are hanged from their halves on being folded, the tibial side burdened with sutures is systematically the weakest point of this reconstruction(50).
The 3ST construct is the format resulting from dividing the length of the semitendinosus in three. It is a valid option in both single-bundle and in double-bundle reconstructions. In the former case, this triple configuration ensures the necessary length of the plasty, though the diameter may be insufficient. Under laboratory conditions, the 3ST format has significantly different mechanical properties depending on the graft preparation method used. In this regard it is essential to bind the three plasty bundles with sutures(55). Under laboratory conditions its behavior both after cyclic loading and in terms of resistance to failure is equivalent to that of the 2ST + 2G model.
Conditioning of the plasty
The ischiotibial tendons used as graft material exhibit viscoelastic behavior (deformation and stress are time dependent)(56). This accounts for two of their characteristics. The first is that the tendon increases in length under constant loading (flow concept). The second is that stress decreases under constant lengthening.
The surgeon applies a degree of initial stress to the plasty during reconstruction of the ACL to limit postoperative knee laxity. The aim is to reduce elongation of the graft after fixation and to improve the preservation of graft tension and rigidity in the immediate postoperative period. However, as a result of the influence of viscoelasticity in the grafts, the initial tension levels of the ischiotibial tendon autografts decrease drastically in the postoperative period (over 50% in an hour)(57,58). The postoperative tension of the graft within the knee is drastically lower than initially, influenced even by the increase in temperature of the plasty (to body temperature) (57). The literature has reported that this is one possible explanation for the difficulties in obtaining a hard anterior compartment end point or objective measurements of physiological laxity in 5-20% of the patients(59,60). Likewise, Handl et al. described that graft stress is a critical factor controlled by the surgeon and which affects the outcome(61).
By managing the rheological properties of the plasty and before its implantation, in order to minimize tension loss, the surgeon may perform different graft manipulations to avoid the rapid decrease in graft tension(62). One option is pre-tightening (static loading on the graft preparation table) before insertion in the bone tunnels. There is no agreement as to the optimum magnitude (in time and force), with values ranging from 44-88 N during 10-30 minutes(63). In any case, pre-tightening of the graft could reduce the amount of elongation, and it has been shown to reduce the postoperative tension loss and rigidity due to viscoelasticity(58).
As mentioned previously, the initial graft stress decreases with cyclic loading, with an increase in knee laxity after 1000-1500 cycles(64). As a consequence of both the viscoelastic behavior of the graft and of the interfaces between the different materials of the construct, they may constitute primary elongation sites(65). Since most of the lengthening occurs in the first cycles after fixation of the plasty(64), repeated flexion and extension of the knee joint (20-30 cycles) allows the length of the graft to reach a stable level(66,67). The greater the length of the graft, the greater the elongation on pre-tightening the entire material; the shorter the length, the earlier the end of elongation will be reached(67).
Length of the tunnel and biological fixation
Definitive fixation of the graft requires its osteointegration via a fibrous interface containing anchoring fibers known as Sharpey fibers. Histological studies suggest that the continuity of the collagen fibers between the graft and the bone gradually increases over time(68,69), and that they are preferentially located around the intraarticular exit from the tunnel(70). Experimental studies have correlated the increase in graft anchoring strength to both time and to the length of the graft within the tunnel(11,71). A short tunnel or an excess of suture material will result in a decrease in tendon-bone interface and would imply a decrease in healing tissue area and thus lesser biological fixation.
Conventionally, a tunnel length of 20-25 mm is recommended(35). Sufficient biological fixation with a 15 mm tunnel has not been demonstrated in humans. The surgical habit of considering a 15 mm femoral tunnel to be sufficient for biological fixation has been established from studies in dogs, and which must be interpreted with caution(72). The sample sizes of the comparator groups are very small: n = 3(72); n = 7(53). Furthermore, the anchoring forces obtained(< 300 N) are clearly lower than the forces which the patient supports under normal conditions during rehabilitation immediately after surgery (300-500 N)(73).
Short tunnels and the presence of suture material reduce the fibrous anchoring interface of the graft, adversely affecting biological fixation(74). The biological incorporation (ligamentization) of plasties with longer configurations (2ST-2G) in comparison with shorter plasties (4ST), as measured by magnetic resonance imaging (MRI), appears to be similar(75).
Conclusions
Isometry is a basic element in reconstruction of the ACL; it protects the graft from excessive distractions, thereby preserving its rheological properties.
The diameter and length of the ischiotibial tendon autografts are limited variables conditioned to harvesting of the tissue.
The literature on the importance of the diameter is more abundant, though plasty diameter and length are inverse variables. Situations of increased risk of repeat rupture, or anthropometric characteristics further away from the average, may lead to the consideration of other reconstruction models different from the single-bundle format.
Plasty configuration has gained polymorphism thanks to the current adjustable fixation devices, seeking to afford the required plasty diameter and length.
Considering the comments of the first section, it is advisable to precondition the ischiotibial tendon graft in both static and dynamic conditions.
Definitive biological fixation of the graft in humans may be influenced by the presence of the graft preparation sutures and the shortness of the tunnels. The conclusions drawn from animal models regarding fixation in short tunnels must be extrapolated with caution to reconstructions in humans.
Figuras
Figure 1. Stress-deformation curve. 1) Initial region (toe region): the curve describes the behavior of the tendons under low deformation (up to 3%), with interweaving of the collagen fibers. 2) Linear region (elastic behavior): the tendon deforms in a linear manner due to intermolecular sliding of the triple helixes of the collagen. If deformation is less than 5%, it proves reversible. 3) Yielding and failure region (plastic behavior).
Figure 2. Flow plot: property of the material implying increasing deformation (creep) over time when the material is exposed to constant stress.
Figure 3. Stress relaxation is a characteristic of a tendon whereby the stress acting upon it ends up decreasing under constant deformation over time.
Figure 4. S-N plot: maximum cyclic stress to which the material is exposed, versus the logarithm of the number N of cycles to the point of rupture. Under dynamic conditions, the materials disrupt according to the number of loading cycles.
Figure 5. The most isometric point of the femur in a right-side knee (with less than 1 mm of change in length) is located 3 mm distal to the posterior margin of the Blumensaat (intercondylar) line(12,13).
Figure 6. Mean graft stress variations. The green line represents the stress variations suffered by the graft during the range of motion of the knee under "isometric behavior" conditions. The red line represents the stress pattern experienced by the graft with anterior location of the femoral tunnel, presenting significantly increased values in the range of motion of 80-120º.
Figure 7. The graft fibers in a right knee cannot simultaneously exhibit anteromedial (blue fibers) and posterolateral behavior (red fibers). Moving the center of the tunnel distal recruits more stressed fibers with posterolateral behavior (stress in extension), though at the expense of reducing the number of fibers available for anteromedial behavior (stress in flexion).
Figure 8. A: Krackow suture; B: "wheat spike" suture to reduce the number of tendon perforations and the amount of suture material.
Información del artículo
Cita bibliográfica
Autores
Iñaki Mediavilla
Servicio de Cirugía Ortopédica y Traumatología. Hospital de Basurto. Bilbao, Bizkaia
Universidad del País Vasco. Bilbao
Mikel Aramberri
Alai Sports Medicine Clinic. Madrid
Servicio de Cirugía Ortopédica y Traumatología. Hospital Universitario Ramón y Cajal. Madrid
Giovanni Tiso
Alai Sports Medicine Clinic. Madrid
Servicio de Cirugía Ortopédica y Traumatología. Hospital Universitario Ramón y Cajal. Madrid
Eric Margalet
Institut Margalet of Arthroscopy. Barcelona
Institut Margalet de Cirugía Artroscòpica. Marbella
Ferrán Abat González
Traumatólogo deportivo, ReSport Clinic Barcelona.
Universidad Blanquerna, Ramon Llull. Barcelona
Servicio de Cirugía Ortopédica y Traumatología. Hospital de la Santa Creu i Sant Pau. Universitat Autònoma de Barcelona
Ethical responsibilities
Conflicts of interest. The authors state that they have no conflicts of interest.
Financial support. This study has received no financial support.
Protection of people and animals. The authors declare that this research has not involved human or animal experimentation.
Data confidentiality. The authors declare that the protocols of their work center referred to the publication of patient information have been followed.
Right to privacy and informed consent. The authors declare that no patient data appear in this article.
Referencias bibliográficas
-
1Chechik O, Amar E, Khashan M, Lador R, Eyal G, Gold A. An international survey on anterior cruciate ligament reconstruction practices. Int Orthop. 2013;37(02):201-6.
-
2Lake SP, Miller KS, Elliott DM, Soslowsky LJ. Tensile properties and fiber alignment of human supraspinatus tendon in the transverse direction demonstrate inhomogeneity, nonlinearity, and regional isotropy. J Biomech. 2010 Mar 3;43(4):727-32.
-
3Johnson GA, Tramaglini DM, Levine RE, Ohno K, Choi NY, Woo SL. Tensile and viscoelastic properties of human patellar tendon. J Orthop Res. 1994;12(6):796-803.
-
4Penner DA, Daniel DM, Wood P, Mishra D. An in vitro study of anterior cruciate ligament graft placement and isometry. Am J Sports Med. 1988 May-Jun;16(3):238-43.
-
5Cohen RE, Hooley CJ, McCrum NG. Viscoelastic creep of collagenous tissue. J Biomech. 1976;9(4):175-84.
-
6Maganaris CN. Tendon conditioning: artefact or property? Proc Biol Sci. 2003 Aug 7;270 Suppl 1(Suppl 1):S39-42.
-
7Amis AA, Dawkins GP. Functional anatomy of the anterior cruciate ligament. Fibre bundle actions related to ligament replacements and injuries. J Bone Joint Surg Br. 1991 Mar;73(2):260-7.
-
8Sidles JA, Larson RV, Garbini JL, Downey DJ, Matsen FA. Ligament length relationships in the moving knee. J Orthop Res. 1988;6:593-610.
-
9Hefzy MS, Grood ES, Noyes FR. Factors affecting the region of most isometric femoral attachments, part II: the anterior cruciate ligament. Am J Sports Med. 1989;17(2):208-16.
-
10Sapega AA, Moyer RA, Schneck C, Komalahiranya N. Testing for isometry during reconstruction of the anterior cruciate ligament. Anatomical and biomechanical considerations. J Bone Joint Surg (Am). 1990;72-B:259-67.
-
11Rodeo SA, Kawamura S ,Kim HJ, Dynybil C, Ying L. Tendon healing in a bone tunnel differs at the tunnel entrance versus the tunnel exit: an effect of graft-tunnel motion? Am J Sports Med. 2006;34(11):1790-800.
-
12Zavras TD, Race A, Bull AM, Amis AA. A comparative study of 'isometric' points for anterior cruciate ligament graft attachment. Knee Surg Sports Traumatol Arthrosc. 2001;9(1):28-33.
-
13Zavras TD, Race A, Amis AA. The effect of femoral attachment location on anterior cruciate ligament reconstruction: graft tension patterns and restoration of normal anterior-posterior laxity patterns. Knee Surg Sports Traumatol Arthrosc. 2005 Mar;13(2):92-100.
-
14Edwards A, Bull AM, Amis AA. The attachments of the anteromedial and posterolateral fibre bundles of the anterior cruciate ligament, part 2: Femoral attachment. Knee Surg Sports Traumatol Arthrosc 2008;16:29-36.
-
15Amis AA. The functions of the fibre bundles of the anterior cruciate ligament in anterior drawer, rotational laxity and the pivot shift. Knee Surg Sports Traumatol Arthrosc. 2012;20:613-20.
-
16Smith JO, Yasen S, Risebury MJ, Wilson AJ. Femoral and tibial tunnel positioning on graft isometry in anterior cruciate ligament reconstruction: a cadaveric study. J Orthop Surg (Hong Kong). 2014;22:318-24.
-
17Kawaguchi Y, Kondo E, Takeda R, Akita K, Yasuda K, Amis AA. The role of fibers in the femoral attachment of the anterior cruciate ligament in resisting tibial displacement. Arthroscopy. 2015;31:435-44.
-
18Iwaki H, Pinskerova V, Freeman MA. Tibiofemoral movement 1: the shapes and relative movements of the femur and tibia in the unloaded cadaver knee. J Bone Joint Surg Br. 2000 Nov;82(8):1189-95.
-
19Ho JY, Gardiner A, Shah V, Steiner ME. Equal kinematics between central anatomic single-bundle and double-bundle anterior cruciate ligament reconstructions. Arthroscopy. 2009;25(5):464-72.
-
20Kondo E, Merican AM, Yasuda K, Amis AA. Biomechanical comparison of anatomic double-bundle, anatomic single-bundle, and nonanatomic single-bundle anterior cruciate ligament reconstructions. Am J Sports Med. 2011 Feb;39(2):279-88.
-
21Abrahams M. Mechanical behaviour of tendon in vitro. A preliminary report. Med Biol Eng. 1967 Sep;5(5):433-43.
-
22Tiamklang T, Sumanont S, Foocharoen T, Laopaiboon M. Double-bundle versus single-bundle reconstruction for anterior cruciate ligament rupture in adults. Cochrane Database Syst Rev. 2012 Nov 14;11(11):CD008413.
-
23Rahr-Wagner L, Thillemann TM, Pedersen AB, Lind MC. Increased risk of revision after anteromedial compared with transtibial drilling of the femoral tunnel during primary anterior cruciate ligament reconstruction: results from the Danish Knee Ligament Reconstruction Register. Arthroscopy. 2013 Jan;29(1):98-105.
-
24Erquicia JI, Gelber PE, Doreste JL, Pelfort X, Abat F, Monllau JC. How to improve the prediction of quadrupled semitendinosus and gracilis autograft sizes with magnetic resonance imaging and ultrasonography. Am J Sports Med. 2013 Aug;41(8):1857-63.
-
25Pérez-Prieto D, Perelli S, Corcoll F, Rojas G, Montiel V, Monllau JC. The vancomycin soaking technique: no differences in autograft re-rupture rate. A comparative study. Int Orthop. 2021 Jun;45(6):1407-11.
-
26Ferretti A, Monaco E, Giannetti S, Caperna L, Luzon D, Conteduca F. A medium to long-term follow-up of ACL reconstruction using double gracilis and semitendinosus grafts. Knee Surg Sports Traumatol Arthrosc. 2011 Mar;19(3):473-8.
-
27Chen L, Cooley V, Rosenberg T. ACL reconstruction with hamstring tendon. Orthop Clin North Am. 2003 Jan;34(1):9-18.
-
28Streich NA, Reichenbacher S, Barié A, Buchner M, Schmitt H. Long-term outcome of anterior cruciate ligament reconstruction with an autologous four-strand semitendinosus tendon autograft. Int Orthop. 2013 Feb;37(2):279-84.
-
29Magnussen RA, Lawrence JT, West RL, Toth AP, Taylor DC, Garrett WE. Graft size and patient age are predictors of early revision after anterior cruciate ligament reconstruction with hamstring autograft. Arthroscopy. 2012 Apr;28(4):526-31.
-
30Conte EJ, Hyatt AE, Gatt CJ Jr, Dhawan A. Hamstring autograft size can be predicted and is a potential risk factor for anterior cruciate ligament reconstruction failure. Arthroscopy. 2014 Jul;30(7):882-90.
-
31Chiang ER, Ma HL, Wang ST, Hung SC, Liu CL, Chen TH. Hamstring graft sizes differ between Chinese and Caucasians. Knee Surg Sports Traumatol Arthrosc. 2012 May;20(5):916-21.
-
32Moghamis I, Abuodeh Y, Darwiche A, Ibrahim T, Al Ateeq Al Dosari M, Ahmed G. Anthropometric correlation with hamstring graft size in anterior cruciate ligament reconstruction among males. Int Orthop. 2020 Mar;44(3):577-84.
-
33Goyal S, Matias N, Pandey V, Acharya K. Are pre-operative anthropometric parameters helpful in predicting length and thickness of quadrupled hamstring graft for ACL reconstruction in adults? A prospective study and literature review. Int Orthop. 2016 Jan;40(1):173-81.
-
34Papastergiou SG, Konstantinidis GA, Natsis K, Papathanasiou E, Koukoulias N, Papadopoulos AG. Adequacy of semitendinosus tendon alone for anterior cruciate ligament reconstruction graft and prediction of hamstring graft size by evaluating simple anthropometric parameters. Anat Res Int. 2012;2012:424158.
-
35Colombet P, Graveleau N. An Anterior Cruciate Ligament Reconstruction Technique With 4-Strand Semitendinosus Grafts, Using Outside-In Tibial Tunnel Drilling and Suspensory Fixation Devices. Arthrosc Tech. 2015 Sep 28;4(5):e507-11.
-
36Pichler W, Tesch NP, Schwantzer G, et al. Differences in length and cross-section of semitendinosus and gracilis tendons and their effect on anterior cruciate ligament reconstruction: a cadaver study. J Bone Joint Surg Br. 2008 Apr;90(4):516-9.
-
37Ramkumar PN, Hadley MD, Jones MH, Farrow LD. Hamstring Autograft in ACL Reconstruction: A 13-Year Predictive Analysis of Anthropometric Factors and Surgeon Trends Relating to Graft Size. Orthop J Sports Med. 2018 Jun 19;6(6):2325967118779788.
-
38Janssen RPA, van der Velden MJF, van den Besselaar M, Reijman M. Prediction of length and diameter of hamstring tendon autografts for knee ligament surgery in Caucasians. Knee Surg Sports Traumatol Arthrosc. 2017 Apr;25(4):1199-204.
-
39Sonnery-Cottet B, Daggett M, Fayard JM, et al. Anterolateral Ligament Expert Group consensus paper on the management of internal rotation and instability of the anterior cruciate ligament - deficient knee. J Orthop Traumatol. 2017 Jun;18(2):91-106.
-
40Parkinson B, Robb C, Thomas M, Thompson P, Spalding T. Factors That Predict Failure in Anatomic Single-Bundle Anterior Cruciate Ligament Reconstruction. Am J Sports Med. 2017 Jun;45(7):1529-36.
-
41Sonnery-Cottet B, Thaunat M, Freychet B, Pupim BH, Murphy CG, Claes S. Outcome of a Combined Anterior Cruciate Ligament and Anterolateral Ligament Reconstruction Technique With a Minimum 2-Year Follow-up. Am J Sports Med. 2015 Jul;43(7):1598-605.
-
42Mediavilla I, Aramberri M, Tiso G, Murillo-González JA. Combined Double Bundle Anterior Cruciate Ligament Reconstruction and Anterolateral Ligament Reconstruction. Arthrosc Tech. 2018 Jul 30;7(8):e881-e886.
-
43Colombet P, Graveleau N, Jambou S. Incorporation of Hamstring Grafts Within the Tibial Tunnel After Anterior Cruciate Ligament Reconstruction: Magnetic Resonance Imaging of Suspensory Fixation Versus Interference Screws. Am J Sports Med. 2016 Nov;44(11):2838-45.
-
44Schurz M, Tiefenboeck TM, Winnisch M, et al. Clinical and Functional Outcome of All-Inside Anterior Cruciate Ligament Reconstruction at a Minimum of 2 Years' Follow-up. Arthroscopy. 2016 Feb;32(2):332-7.
-
45Colombet P, Saffarini M, Bouguennec N. Clinical and Functional Outcomes of Anterior Cruciate Ligament Reconstruction at a Minimum of 2 Years Using Adjustable Suspensory Fixation in Both the Femur and Tibia: A Prospective Study. Orthop J Sports Med. 2018 Oct 22;6(10):2325967118804128.
-
46Brown C. Five- and Six-Strand Hamstring Tendon Graft Preparation for Single-Bundle Hamstring Anterior Cruciate Ligament Reconstruction. En: Prodromos CC. The Anterior Cruciate Ligament. Elsevier; 2018.
-
47Krackow KA. The Krackow suture: how, when, and why. Orthopedics. 2008 Sep;31(9):931-3.
-
48Barber FA, Howard MS, Piccirillo J, Spenciner DB. A Biomechanical Comparison of Six Suture Configurations for Soft Tissue-Based Graft Traction and Fixation. Arthroscopy. 2019 Apr;35(4):1163-9.
-
49Wang RY, Arciero RA, Obopilwe E, Mazzocca AD. A comparison of structural and mechanical properties of tubularized and native semitendinosus graft. Am J Sports Med. 2010 Jun;38(6):1246-9.
-
50Wichern CR, Skoglund KC, O'Sullivan JG, et al. A biomechanical comparison of all-inside cruciate ligament graft preparation techniques. J Exp Orthop. 2018 Oct 10;5(1):42.
-
51Theopold J, Schleifenbaum S, Georgi A, et al. The single-suture technique for anterior cruciate ligament graft preparation provides similar stability as a three-suture technique: a biomechanical in vitro study in a porcine model. Arch Orthop Trauma Surg. 2020 Apr;140(4):511-6.
-
52Bowes J, Mohamed N, Baptiste JJ, Westover L, Hui C, Sommerfeldt M. Biomechanical Comparison of Graft Preparation Techniques for All-Inside Anterior Cruciate Ligament Reconstruction. Orthop J Sports Med. 2020 Jul 24;8(7):2325967120938039.
-
53Qi L, Chang C, Jian L, Xin T, Gang Z. Effect of varying the length of soft-tissue grafts in the tibial tunnel in a canine anterior cruciate ligament reconstruction model. Arthroscopy. 2011;27:825-33.
-
54Chen H, Liu H, Chen L. Patellar Tendon Versus 4-Strand Semitendinosus and Gracilis Autografts for Anterior Cruciate Ligament Reconstruction: A Meta-analysis of Randomized Controlled Trials With Mid- to Long-Term Follow-Up. Arthroscopy. 2020 Aug;36(8):2279-91.e8.
-
55Geethan I, Santhosh Sahanand K, Ashwin Vijay PR, Rajan DV. Mechanical assessment of tripled hamstring tendon graft when using suspensory fixation for cruciate ligament reconstruction. J Exp Orthop. 2018 Nov 27;5(1):48.
-
56Rowden NJ, Sher D, Rogers GJ, Schindhelm K. Anterior cruciate ligament graft fixation. Initial comparison of patellar tendon and semitendinosus autografts in young fresh cadavers. Am J Sports Med. 1997 Jul-Aug;25(4):472-8.
-
57Ciccone WJ 2nd, Bratton DR, Weinstein DM, Elias JJ. Viscoelasticity and temperature variations decrease tension and stiffness of hamstring tendon grafts following anterior cruciate ligament reconstruction. J Bone Joint Surg Am. 2006 May;88(5):1071-8.
-
58Elias JJ, Kilambi S, Ciccone WJ 2nd. Tension level during preconditioning influences hamstring tendon graft properties. Am J Sports Med. 2009 Feb;37(2):334-8.
-
59Brandsson S, Faxén E, Kartus J, Jerre R, Eriksson BI, Karlsson J. A prospective four- to seven-year follow-up after arthroscopic anterior cruciate ligament reconstruction. Scand J Med Sci Sports. 2001 Feb;11(1):23-7.
-
60Labs K, Perka C, Schneider F. The biological and biomechanical effect of different graft tensioning in anterior cruciate ligament reconstruction: an experimental study. Arch Orthop Trauma Surg. 2002;122:193-9.
-
61Handl M, Drzík M, Cerulli G, et al. Reconstruction of the anterior cruciate ligament: dynamic strain evaluation of the graft. Knee Surg Sports Traumatol Arthrosc. 2007 Mar;15(3):233-41.
-
62Pilia M, Murray M, Guda T, Heckman M, Appleford M. Pretensioning of Soft Tissue Grafts in Anterior Cruciate Ligament Reconstruction. Orthopedics. 2015 Jul 1;38(7):e582-7.
-
63Jisa KA, Williams BT, Jaglowski JR, Turnbull TL, LaPrade RF, Wijdicks CA. Lack of consensus regarding pretensioning and preconditioning protocols for soft tissue graft reconstruction of the anterior cruciate ligament. Knee Surg Sports Traumatol Arthrosc. 2016 Sep;24(9):2884-91.
-
64Arnold MP, Lie DT, Verdonschot N, de Graaf R, Amis AA, van Kampen A. The remains of anterior cruciate ligament graft tension after cyclic knee motion. Am J Sports Med. 2005 Apr;33(4):536-42.
-
65Höher J, Scheffler SU, Withrow JD, et al. Mechanical behavior of two hamstring graft constructs for reconstruction of the anterior cruciate ligament. J Orthop Res. 2000 May;18(3):456-61.
-
66Jiang D, Ao YF, Jiao C, et al. The effect of cyclic knee motion on the elongation of four-strand hamstring autograft in anterior cruciate ligament reconstruction: an in-situ pilot study. BMC Musculoskelet Disord. 2019 Jul 9;20(1):321.
-
67Kawano CT, de Moraes Barros Fucs PM, Severino NR. Pretensioning of quadruple flexor tendon grafts in two types of femoral fixation: quasi-randomised controlled pilot study. Int Orthop. 2011 Apr;35(4):521-7.
-
68Liu SH, Panossian V, al-Shaikh R. Morphology and matrix composition during early tendon to bone healing. Clin Orthop Relat Res. 1997;339:253-60.
-
69Beynnon BD, Johnson RJ, Abate JA, Fleming BC, Nichols CE. Treatment of anterior cruciate ligament injuries, part I. Am J Sports Med. 2005;33:1579-602.
-
70Shoemaker SC, Rechl J, Campbell P, Kram HB, Sánchez M. Effects of fibrin sealant on incorporation of autograft and xenograft tendons within bone tunnels. Am J Sports Med. 1989;17:318-24.
-
71Greis PE, Burks RT, Bachus K, Luker MG. The influence of tendon length and fit on the strength of a ten- don-bone tunnel complex: A biomechanical and histologic study in the dog. Am J Sports Med. 2001;29:493-7.
-
72Yamazaki S, Yasuda K, Tomita F, et al. The effect of intraosseous graft length on tendon-bone healing in anterior cruciate ligament reconstruction using flexor tendon. Knee Surg Sports Traumatol Arthrosc. 2006;14(11):1086-93x.
-
73Shelburne KB, Pandy MG, Anderson FC, Torry MR. Pattern of anterior cruciate ligament force in normal walking. J Biomech. 2004 Jun;37(6):797-805.
-
74Barbosa NC, Guerra-Pinto F, Cabeleira C, Beja da Costa P. Hamstring Graft Biological Preparation for Anterior Cruciate Ligament Reconstruction. Arthrosc Tech. 2017 Feb 27;6(1):e245-e248.
-
75Cavaignac E, Marot V, Faruch M, et al. Hamstring Graft Incorporation According to the Length of the Graft Inside Tunnels. Am J Sports Med. 2018 Feb;46(2):348-56.
Descargar artículo:

Licencia:
Este contenido es de acceso abierto (Open-Access) y se ha distribuido bajo los términos de la licencia Creative Commons CC BY-NC-ND (Reconocimiento-NoComercial-SinObraDerivada 4.0 Internacional) que permite usar, distribuir y reproducir en cualquier medio siempre que se citen a los autores y no se utilice para fines comerciales ni para hacer obras derivadas.
Comparte este contenido
En esta edición
- The COVID hangover
- Results of the arthroscopic treatment of acute bony Bankart lesion using the original Porcellini-Sugaya technique and the double-row modified procedure
- Assessment of tibial translation in experimental models of anterior cruciate ligament reconstructions: is dual tibial fixation indicated?
- Learning curve in the arthroscopic Latarjet technique
- Clinical outcomes of the anteromedial technique versus the transtibial technique in reconstruction of the anterior cruciate ligament: a systematic review and meta-analysis of randomized clinical trials
- Basic sciences in reconstruction of the anterior cruciate ligament with the ischiotibial tendons (a narrative review)
- Arthroscopic hip surgery in femoroacetabular impingement
- Circumferential 270º reconstruction of the hip labrum through arthroscopy with knotless tensionable technology Surgical technique
- Arthroscopic capsule reconstruction with dermal mesh plus segmental labral reconstruction with tibialis anterior allograft in recurrent postsurgical hip microinstability
- Synthetic polyethylene mesh augmentation for chronic patellar tendon rupture repair
- The Giant’s Causeway “inside the shoulder”
Más en PUBMED
Más en Google Scholar
Más en ORCID
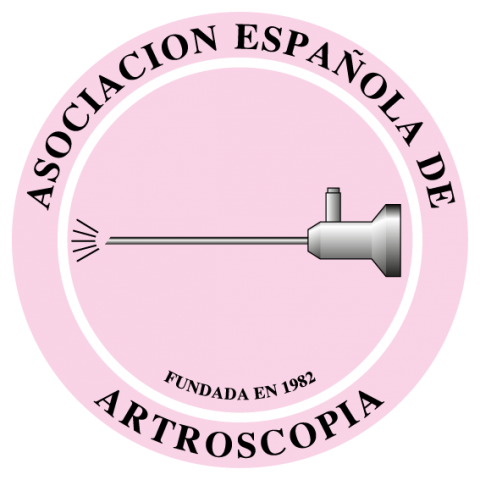

Revista Española de Artroscopia y Cirugía Articular está distribuida bajo una licencia de Creative Commons Reconocimiento-NoComercial-SinObraDerivada 4.0 Internacional.